A look back from year 2050 – on what we thought we once knew.
Lorenzo Kristov is principal for market and infrastructure policy at the California Independent System Operator. The ideas discussed in this article are the views and opinions of the author and do not reflect policies or positions of the California Independent System Operator.
In hindsight, from our vantage point here in the year 2050, we can see now how far off the mark we were back in 2015.
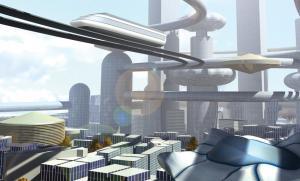
The so-called 'disruptive' challenges to the electric system, which seemed so chaotic during the first two decades of this century, have now run their course. Today, some 35 years later, those challenges have converged into a new industry model - but not the one we foresaw back in 2015.
But we should have understood, even back then. Given the broad consensus on the forces at play during those years, the 'integrated decentralized' paradigm that prevails now in 2050 could not have been more obvious. Why couldn't we figure out where the industry was heading?
On the other hand, however, it is a bit surprising that everything happened so fast. The basic framework of the new paradigm was widely accepted early on - certainly by 2030, when its implementation well was underway - with many of its structural elements already in place in many parts of the country.
Which brings us back to the present, at mid-century. And so this article will provide a retrospective snapshot of the U.S. electric system - how it looked in 2015, how it had changed so much by 2030, and how the drivers of change that were so rampant during the twenty-teens converged eventually to form a coherent, well-functioning, and brand-new electric system.
The Drivers of Change
The main drivers of change had been gaining momentum well before the 21st century began.
First came the undeniable need to end the adverse environmental impacts of our energy practices. Second, we saw the adoption of clean-energy policies and mandates. Third, customers and local jurisdictions made clear their desires for 'resilience' and greater control of their energy futures. Fourth came the falling costs and increasing capabilities of clean, small-scale technologies - particularly solar and storage. And lastly, we saw the emergence of innovative business models designed to facilitate all of the above.
By the twenty-teens the industry had recognized that these change drivers were not just passing fads. Rather, they would dramatically alter how electricity was produced, distributed, and consumed. At the same time, efforts to envision and design a very different grid of the future were not getting very far. Most industry experts seemed to expect either a gradual process of change by incremental adoption and integration of new technologies, or a continuing disruptive process that would not settle into a new structure for a very long time.
The first significant shift - the one that set the industry on a clear path to a new paradigm - was the recognition that distribution-connected energy resources (DER) were not just going to be a part of the future electric system, they were going to dominate it. Until that insight became widespread, around 2015, most industry professionals and entrepreneurs expected that the then-current wholesale market, based upon congestion, flows, and resource location with the bulk transmission grid - would remain the dominant core of the power system. In accord with that assumption, these market players had focused most of their efforts on ways to make distributed resources look and behave like central station power plants and to partake of established revenue streams from wholesale energy markets and from supplying capacity to meet resource adequacy rules. Federal and state regulators at the time reinforced this approach by encouraging both sides - the distributed resource suppliers as well as the utility buyers and wholesale market operators - to find ways to treat such resources comparably to conventional power plants and include them in their procurement and economic dispatch procedures.
At the same time, however, others recognized that DERs would come to dominate the future electricity system. That realization enabled the leading edge of the industry to venture outside the central-station paradigm and coalesce around the model of an 'integrated decentralized' system. The 'decentralized' aspect referred to the emergence of local, largely self-reliant distribution-based systems that could supply most of their power needs from local resources and, when necessary, could island. The 'integrated' aspect reflected the fact that these local systems could remain interconnected to the regional transmission grid most of the time to interact with the wholesale markets, and would use their islanding capability only when needed, such as to deal with a major disruption to the larger system.
With this new structural model in view, the industry focus shifted to designing local-scale electric systems, which included micro-grids to serve large complex facilities, such as university campuses and major medical centers, as well as 'community micro-grids' comprised of all the diverse customers and distributed resources in a local distribution area.
The System by 2030
The grid by 2030 was clearly on the way to becoming an interconnected federation of local power systems, with "local" defined as the electrical footprint served by a single transmission-distribution substation and typically aligned with or a part of the political jurisdiction responsible for municipal services in the area. In many places smaller "self-optimizing" systems - campus micro-grids, even individual buildings - were nested within the local area. In some areas the existing utility distribution companies became distribution system operators (DSOs) for the local areas that comprised their traditional service territories. In other areas new DSO companies entered the scene to partner with local jurisdictions and perform operations and planning functions to enable cities, counties, water authorities, and other local agencies to design and implement their own customized portfolios of energy services and resources.
Most of these DSO-operated local power systems developed islanding capability, but under normal conditions they remained connected to the transmission grid to enable DERs and customers to transact with the wholesale markets. A key operating principle guiding the design of DSO operation was to manage the inter-temporal variability of DER and end-use customers close to the source as much as possible, consistent with economic and operational efficiency. With the recognition that real-time variability management was becoming a major cost driver of reliable operation, regulators approved rate structures and financial incentives at all levels of the system to reinforce this principle.
At the level of the wholesale market (run by the independent system operator, or ISO), we saw that DSO settlements with the ISO reflected the inter-temporal variability of the net energy flow across the transmission-distribution substation. The more the DSO could manage this variability locally, the less it would have to pay the grid operator (ISO) for this service. Grid-connected generators and import/export transactions also faced such changes in their compensation structures. Analogously, a DSO's charges for distribution service also reflected each distribution customer's variability. And in this context, the DSO's "customers" now included all types of interconnected DERs, as well as energy end-users. As a result, each facility operator connected to the transmission or distribution system faced the economic decision whether to pay to export its variability to the system or install storage combined with power electronics and advanced controls to manage it on-site. This one innovation in the pricing of transmission and distribution service stimulated advances in the cost-effectiveness of storage to the point where it became a ubiquitous grid element, as fundamental as transformers and circuit breakers.
Another factor that encouraged local management of variability and stimulated growth of energy storage at all scales was the increasing frequency of negative wholesale prices. Renewable portfolio standards and policies to control greenhouse gases had led to high penetrations of variable renewable generation (wind and solar). But that created more frequent periods of excess supply, sometimes creating local congestion in generation pockets, but more often creating excess generation supply on the system as a whole, causing prices sometimes to turn negative. For the renewable generators, negative prices quickly translated to decisions to invest in energy storage, either located right on-site or shared with other generators in the same electrical area, to absorb excess energy when spot prices went negative and then supply it when prices rose again.
Policy makers quickly saw another message in frequent negative prices - namely, the opportunity to advance environmental policy goals by growing new types of electricity demand to use the abundant and clean solar and wind power. By 2020 California was definitively on a path to expand both electric and hydrogen vehicles, using renewable energy to power daytime vehicle charging at work places and also to power water electrolysis to create hydrogen, resulting in an efficient synergy of energy storage with transportation. Water desalination - another large, flexible consumer of electricity - as well as new types of utility-scale storage served also to to help keep supply and demand in balance.
All of these developments were traceable to the incentives created by pricing transmission and distribution service based on inter-temporal variability and negative spot prices in times of excess generation. Yet perhaps the most notable of these outcomes was this: the operational challenge of integrating variable renewables into the grid - a challenge that was believed so great back in the early twenty-teens - turned out eventually to be much less severe than expected and more readily manageable with new forms of grid control. After about 2020 the operational impacts of renewables integration were no longer of great concern, even in areas such as California where much of the fossil fleet had retired.
Partnerships with Local Jurisdictions
By the early twenty-teens many cities and counties developed their own climate action plans. Some were responding to state requirements; others were simply frustrated by the absence of any effective national policy to address worsening environmental impacts.
Most of these local climate action plans included electricity-related elements: energy efficiency, demand response, zero-net-energy programs, municipal vehicle-charging installations, and "solar gardens" where a solar developer would construct a local generating facility and sell energy directly to residential, commercial and municipal end users.
At first these local initiatives were not expected to have much of a role in shaping the future grid. But by 2020, it became apparent that their impact would indeed prove formidable. This shift arose from the recognition by most parties - including developers and vendors of the new technologies, the utility distribution companies, and the state policy makers and regulators - that the transformation of energy required to achieve environmental targets would necessitate new partnerships of all these parties with local communities and governmental entities.
Several factors contributed to the formation of such partnerships. For one, the California legislature, prompted by the growing DER expansion, directed the distribution utilities to file distribution resources plans (DRPs) by mid-2015. Among other things, these plans needed to include methods for determining optimal locations for deployment of DERs. Such methods would reflect the integration capacity of their existing systems, the locational value of the grid services that DERs could provide, and the nature and cost of distribution system upgrades that would be needed.
A public working group, which was formed to help develop the details of the required planning methods, came to realize that the criteria for "optimal" had to come from two complementary perspectives - a system perspective and a customer perspective.
On one hand, utilities could assess the local integration capacities of their systems and the value of DER-provided services to the power system, and these elements would provide locational incentives and revenue opportunities to DER developers. On the other hand, however, the growth of DERs was also driven largely by the desires of end-use customers and local jurisdictions to have greater say in their energy futures and reduce their carbon footprints. And energy services firms and DER developers stood ready to fulfill those desires.
And now we come to the nub of it all - the traditional utility business model.
Starting around 2010, many industry experts were predicting that "disruptive" distributed technologies and rate incentives such as feed-in tariffs and net energy metering would so drastically shrink sales volumes that rates for remaining customers would spiral out of control, prompting even more defection. One obvious immediate response (proffered by many of the utilities) was to seek to expand the scope of their regulated activities to include rate-based investment in and ownership of DERs, including behind-the-meter residential rooftop solar and battery configurations, electric-vehicle charging stations, and stand-alone storage and renewable generation installed on the distribution system.
Advances along this route proved both slow and piecemeal in many states. Policymakers voiced concern about stifling innovation in the open competitive market. But while regulators and stakeholders debated and deliberated the merits of allowing utilities to enter various competitive arenas under the regulatory umbrella, no one questioned the natural monopoly nature of the distribution wires business.
Yes, it was clear that DERs would make traditional distribution operations and planning inadequate. DERs also would require new investment in electrical and communications infrastructure and in workforce development to manage the new system. And many customers eyed micro-grids and even larger and more mixed local configurations capable of islanding. Nevertheless, most end users and local systems preferred to stay connected to the grid under all but emergency conditions, and to participate in local and wholesale market transactions.
Thus, by the late twenty-teens, most major utilities planned to play a central and viable role in the 21st century.
But to stay central and viable utilities would have to become full-service distribution wires companies, with or without forays into the more competitive arenas. As such, it was a natural next step to partner with local jurisdictions to gain flexibility in choosing preferred supply sources, to enact energy efficiency and demand response programs, and to develop DER types within their footprints that best fit their residential and commercial customer bases.
Moreover, policymakers and regulators readily saw that combining energy and environmental leadership and innovation at the local level, supported by modernizing the distribution wires utility for the high-DER world, would provide an effective framework to achieve the state's policy goals efficiently and quickly.
DSOs and Imbalance Markets
In many areas, particularly in the western United States where by 2015 there were still no ISOs or RTOs (regional transmission organizations) outside of California, more DSOs emerged with enhanced capabilities to help coordinate distributed energy resources within their local areas. These new 'enhanced' DSOs would manage variability in energy flows over the transmission-distribution interfaces, and even operate local markets for energy and distribution system services. They arose also in parallel with the expansion of real-time energy imbalance markets across most of the West. These new real-time imbalance markets achieved great efficiencies in integrating variable renewable resources, sharing of operating reserves (especially flexible capacity), and increasing capacity factors of transmission utilization by reducing congestion and unscheduled loop flows.
It quickly became apparent, however, that with the expanded geographic footprint of a real-time imbalance market, direct participation by vast numbers of DERs at the transmission-distribution interfaces would compromise much of the desired efficiency. At the same time, the operational benefits of DER were well recognized. Neither the imbalance market operators nor the participating balancing areas wished to exclude DERs from participating. The enhanced DSOs solved this dilemma by aggregating the DERs into a single composite "resource" within each local distribution area (i.e., the area representing the circuits below each transmission-distribution substation). These aggregated DERs would bid into and be dispatched by the operator of the imbalance market.
Thus, instead of the imbalance market seeing and dispatching thousands of DERs at a given interface (or even just a few dozen complex aggregated "virtual" resources), and instead of challenging the DSO to ensure reliability on the distribution system even as these individual DERs responded independently to their dispatch instructions, the market operator now would see only a single DSO-aggregated resource at the interface. And in response to a dispatch of that one single aggregated resource, the DSO would be able to optimize the DERs in the area to comply with the dispatch instruction. These operating arrangements proved so efficient that by the mid twenty-twenties there were real-time imbalance markets covering the entire western U.S., with enhanced DSOs aggregating DERs for wholesale market bidding in nearly all of the DER-rich local areas. And so evolved the new electric system known as the 'integrated decentralized' paradigm.
Another societal benefit of the western imbalance markets with enhanced DSOs was the ability to access renewables-rich areas in the West to supply western load centers with very little investment in new transmission capacity. Since the early part of the century there had been keen interest in tapping the high-quality wind resources in the northern states and solar resources in the Southwest, but most industry experts expected that new transmission capacity would be required - at a substantial cost - to move energy from these locales to the major load centers. A welcome unintended consequence of the expansion of real-time imbalance markets across the west, combined with the proliferation of DER and the resulting reduction in flows on the transmission system, was the capacity of the existing grid to access these more remote high-quality renewable energy resources without significant new transmission investment.
The Luxury of Hindsight
The new integrated decentralized electric system paradigm, which had pretty well taken hold by 2030, was only dimly perceived by 2015. That was due mainly to the sheer numbers of distinct, partially overlapping proceedings, initiatives and venues, both national and state-specific, in which the impacts of the forces of change were being analyzed and new policies debated. Moreover there was a pronounced tendency - one only natural in an industry both crucial to the well-being of society as a whole and yet subject to the unforgiving laws of physics - to try to manage change in small increments and avoid major departures from the status quo. What actually emerged, as described in this article, was a pretty dramatic departure from the status quo.
From our current vantage point in 2050, it is easy to see how the forces at play in 2015 culminated in the new dominant paradigm by 2030. In hindsight we can see clearly how certain key insights acted like the final grains of a precipitate added to an already concentrated solution to trigger a transformation. And in this case that transformation looms large; it's the realization that DERs would dominate the future rather than simply lurk at the margin.
This transformation sparked a host of needs: to employ cost-causation principles to charge for transmission and distribution services based on inter-temporal volatility; to recognize the distribution wires business as an attractive and viable future for utilities; to acknowledge the bottom-up nature of the industry, with customers and local jurisdictions wanting to control their own energy futures; and to unleash the technologies needed to make it happen.
The rest, as they say, is history.
Lead image © Denisgo | Dreamstime.com