GHG reduction via residential electricity ratemaking.
Ren Orans (ren@ethree.com) and C.K. Woo are partners at Energy & Environmental Economics Inc. (E3), based in San Francisco, Calif. Michael King and William Morrow are senior consultants with the firm.
On the campaign trail, then-Senator Obama made ambitious statements regarding renewable energy investment and greenhouse-gas (GHG) reduction goals. For example, in October 2007, Senator Obama announced plans to, if elected president, reduce emissions to 1990 levels by 2020 and 80 percent below 1990 levels by 2050.1 Achieving such ambitious goals will require major changes on many fronts. In the electric power sector, an essential component of significant GHG reduction is energy efficiency.
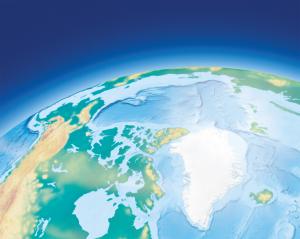
In the arena of electricity efficiency, much attention has been given to building codes and weatherization, efficient lighting and appliance standards, and other measures that can be undertaken by businesses and households, often with incentives from the local electric utility. Rate design has received less attention. However, building on a survey the authors performed for BC Hydro in its 2008 residential rate-design application,2 a study by the authors suggests that rate design—in particular residential inclining block rates—can help achieve GHG-reduction goals. The same opportunity does not exist for time-varying rates.