Opportunity for advancement or exercise in futility?
Mani Vadari (vadari@battelle.org) is vice president of energy infrastructure at Battelle Energy Technologies.
With the U.N. Climate Change Conference in Copenhagen now over, the electric utility industry is under an ever-expanding microscope and is being confronted by a wide array of global energy concerns, a result of political and economic policies targeting climate change. While the Copenhagen Conference might not have been the success that some hoped, it has caused intensified scrutiny. Many of the concerns addressed at the conference have created the most significant impetus for change the industry ever has seen. The subsequent alignment of worries among many politicians, regulators, and customers has renewed concerns that ultimately should result in new legislation and regulations aimed at combating climate change.
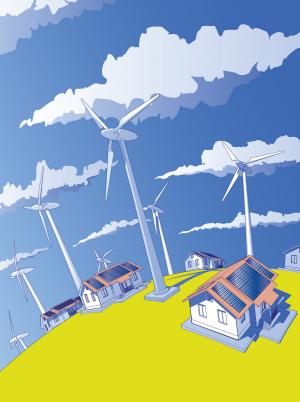
An important concern that will need to be addressed by the industry as it tries to combat climate change is the incorporation of new sources of renewable energy (e.g., wind and solar) into the electric grid. Integrating these new sources of energy will result in a variety of positive outcomes, including the reduction of carbon emissions, the curbing of reliance on foreign sources of energy, and the creation of new green sector jobs that have become a constant topic of discussion in the current economic and political climate.