Smart solutions for distributed renewables.
Kevin Cornish is an executive consultant with Enspiria Solutions Inc., a Black & Veatch company.
The goal of implementing a distribution management system (DMS) is to upgrade isolated, hands-on grid management processes into an interconnected and automated platform. This technology is transforming the way utilities operate distribution networks, and setting the industry on a path toward seamless integration of distributed resources—both supply and demand.
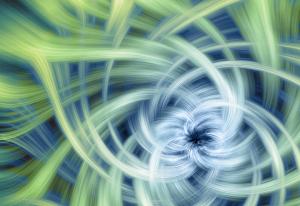
Suppose a utility’s distribution management system detects a dangerous voltage fluctuation on a low-level feeder sometime around mid-day and opts to shed load to fix the problem, but then the operator discovers that the customer in question was operating banks of solar PV on warehouse roofs. You thought you were shedding load (and you were), but you also were shedding a fully dispatched generation supply. Now what?