Someone Else’s Problem Field
Charles Bayless is a retired utility executive having served as chief executive officer of Illinois Power and of Tucson Electric. He was also President of the West Virginia University Institute of Technology, and is a board member of several organizations including the Climate Institute.
To many, especially several of our policy makers, climate change and ocean acidification seem to be perpetually cloaked in a Someone Else’s Problem Field.
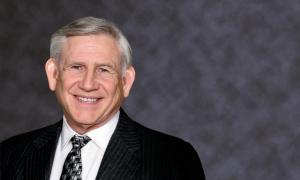
The term was defined by Douglas Adams in his 1982 book Life, the Universe and Everything. Adams was the creator of the comedy sci-fi series Hitchhiker’s Guide to the Galaxy.
Cloaked in such a field, they are effectively invisible. Year after year, scientists warn of the dangers. We pay lip service to them, and our carbon dioxide levels relentlessly increase.
We must realize that the thirty to forty percent renewable targets that many have set are totally inadequate to halt our steady descent into a “hellish future.” To avoid this future will require renewable levels of eighty to ninety percent, and international cooperation.
Achieving these levels will require continued technological progress not only in renewable sources. But also in the platform on which they operate and which enables them, the electric grid.
What we are currently doing, by concentrating on renewable sources to the exclusion of the grid, is akin to airplane manufacturers developing only new engines after World War II. Today we would have modern high-bypass engines on DC-3’s.
To develop the grid, we must make changes in the design of the market for electricity.
An externality is a cost or benefit cloaked in a Someone Else’s Problem Field, invisible to many market participants. A more formal economic definition is a cost or benefit attributable to a transaction in which cost or benefit is imposed on a third person. This person is not a party to the transaction. No cost or benefit market exists.
A good example of an externality is pollution. A power plant generates electricity, which it sells to its customers. The pollution from this transaction however, is felt by others, and no market exists for the pollution. The important point is that no market exists for an externality. The market, by definition, cannot handle externalities.
Externalities abound in the generation of renewable energy. A customer installs rooftop solar and uses net metering to buy or sell to the local utility. As far as the customer and the installer are concerned, that is the entire transaction.
But the installation imposes externality costs on the grid in the form of balancing costs, VAR (volt-amp reactance) support, reserve requirements, etc. Unless these externality costs are internalized by establishing a market for them, the market cannot optimize the system.
Different types of renewables impose different externality costs, and after reviewing the multitude of renewable options, we have two choices. We can internalize all of the costs, carbon, reserves, VAR support, balancing costs, transmission etc., and let the market work.
Or we can return to regulation and use the least-cost planning concept, where regulators look at the total costs of each renewable scheme and pick winners and losers. I vastly prefer the market. But the market cannot work as currently configured, since it does not recognize externality costs.
Let’s now look at just two interrelated factors in system reliability, both of which impose externality costs on the grid, ACE and Frequency Bias. Let’s also look at how renewables change the costs for this service.
The electric grid is like a large lake. Thousands of plants are pouring water into the lake. Millions of customers are taking water out of spigots on the bottom. At the same time, companies are buying and selling across the grid.
How do we keep track of who is using what power? The simple answer is, we don’t. We make no attempt to track which power goes where, because we can’t. But we must keep the grid in balance, as at any instance sources must be equal to uses. To achieve this balance, we utilize a concept known as Area Control Error, ACE.
ACE is the difference between the electricity each utility is putting into the grid through generation or purchases, and the amount we are taking out either through load, losses or sales.
We don’t actually calculate ACE by subtracting uses from sources. Instead, we use a simple method that has the advantage of each measurement being verified by another utility.
Every utility is a control (balancing) area, or is a part of one. That control area will be connected to other control areas by tie-lines. These lines are accurately metered at the interconnection point by both utilities.
If a utility is neither buying nor selling outside its control area, its net tie-line flows should be zero. Net tie-line flow is simply the sum of all flows into and out of the system.
If there is a net outflow of two megawatts, then the utility is generating two megawatts too much. The utility should reduce its generation by two megawatts.
If there is a net inflow of three megawatts, then the utility is short three megawatts. It must increase generation by three megawatts.
Now assume the utility is selling fifty megawatts to another utility. Its net tie-line flows should be fifty megawatts out of the system. If they are not fifty megawatts, the utility must compensate.
Thus every utility measures their tie-line flows and keeps their actual net flows equal to their scheduled flows. The overall result is that the entire system is in balance.
What do we do if ACE is not equal to zero? How do we achieve the second–to-second balancing required?
For small ACE errors, the error is corrected by one plant on AGC, automatic generation control. The AGC plant receives its control input from the ACE signal.
If ACE is minus two megawatts, the AGC plant increases its output by two megawatts. This brings ACE back to zero.
If the ACE signal is plus one megawatt, the plant then decreases its output by one megawatt to bring ACE back to zero. For larger errors, additional plants need to increase or decrease output, or be brought on-line.
There is a further consideration in ACE, one usually reserved for geeky system engineers. But it’s a very important consideration for system stability: frequency bias.
Generators and motors are basically the same thing. They share many of the same characteristics. If more load is put on most motors, they slow down. If more load is put on a generator, it also slows down, resulting in its output frequency decreasing.
Assume now that a thousand megawatt plant trips off-line in Western Colorado. Then the other plants in the Western Interconnection will pick up the extra load and slow down. The frequency in the entire system will decrease, as everyone is tied together.
Everyone has the same frequency. This does not include the Eastern or Texas grids. They are separate grids, and are only connected to the western U.S. and each other through direct current ties.
Two things of major importance happen as frequency decreases at all western utilities. First, governors on generators (analogous to a speed control on a car), throughout the west, automatically try to restore the system frequency by increasing output.
Second, many loads, particularly motor loads, throughout the west, will decrease as frequency decreases. This combination of increased generation and decreased loads will compensate for the lost unit. Then a new system-wide equilibrium can be reached. But it will be at a lower frequency.
The overall grid may again be in balance at this lower frequency. But individual utilities, other than the Colorado utility where the trip occurred, are now seeing a large positive ACE.
Their generation is up and loads are down, resulting in a large outflow. Power is now flowing outward from every western utility to aid the utility where the outage occurred.
If every utility just looked at the first term of the ACE Equation, net tie-line flows, they would decrease their generation to rebalance their control area. This would further decrease frequency, leading to more outflows and more generation decrease. And it would result in a very quick downward spiral to a blackout.
Fortunately, we have a second term in the ACE equation. It’s a term called frequency bias, reflecting how much outflow a utility should expect for a given frequency decrease.
The frequency bias for any given frequency at each utility is derived from a formula based on the actual outflows from past frequency excursions. It approximates how much of an outflow the utility should have at any given frequency.
For instance, the formula may predict that at 59.96 hertz you should see an outflow of thirty-five megawatts. The utility, actually the utility’s ACE computer, just ignores thirty-five megawatts of outflow if the frequency is 59.96 hertz. Someone is in trouble.
Thus if actual tie-line outflows at a utility were forty megawatts, they should reduce by five megawatts to have a net outflow of thirty-five megawatts. As different generators and different motor loads are on-line at the utility during each outage, the frequency bias is not exact but a best estimate, based on curve-fitting techniques using past outages as the input.
The contribution that other utilities make to stability by allowing net outflows falls under the concept of inadvertent interchange, and must be paid back by the Colorado utility in accordance with NERC rules. This one-for-all concept allows us to have fifteen minutes to bring our ACE back to zero.
If each utility had to have the ability to bring its ACE back to zero without help, we would need far more frequency reserves. And we probably would not be able to avoid frequency collapse.
Further, the one-for-all aspect of frequency bias is what allows us to build large units. If we didn’t have the support offered by others, even large utilities would be unable to build a thousand megawatt unit.
As the Colorado utility starts to bring up reserve generation, frequency will increase. Governor-operated units will then decrease their output. Motor loads will pick up. Each utility’s frequency bias will decrease as the system comes back into balance.
To round out the frequency equation, one must also look at generator inertia. Generator inertia is much like the momentum of a vehicle.
If a constant force is applied to a Volkswagen, and to a fully loaded semi-truck, the truck will slow down much faster. The same is true for a power system. The rate at which frequency initially declines is a function of the rotating mass of the generators on-line at the time.
Over the last decade we have continually lost generator inertia in most areas due to the switch from high-inertia units such as coal, to low-inertia units such as gas turbines and renewables.
This decline means that we need other forms of inertia such as flywheels, or other forms of energy injection such as batteries, to preserve the same rate of frequency decline.
In order for the market to achieve an optimum outcome, each provider (including renewable providers) should be responsible for providing their share of the frequency response and ACE requirements, either directly or through purchase. This is necessary for the market to make correct choices.
As renewable units are more variable, they lead to larger ACE measurements. The host utility must compensate for these at a cost. They also lead to larger frequency variations, which also have a cost. Further, different renewable units may impose different costs. These must be taken into account in picking the optimum system.
For example, a unit may be cheaper on a per kilowatt-hour basis. However, if it requires significantly more balancing services and frequency support, it may be cheaper from a societal point of view to build another unit. This new unit would have a slightly higher kilowatt-hour cost, with lower balancing requirements.
For the market to make the correct decision, it must see all costs including carbon costs. We are in a very dangerous position because we have ignored the externalities of carbon. We will make further bad decisions if we ignore the externalities of renewables.
Europe provides an example of where we should go. Currently in most European countries, generators are responsible for the costs of any deviations from their scheduled output. They also pay the costs of any required balancing energy.
If the deviations are opposite to grid needs, then they must also pay a penalty price. For instance, if the grid is in an under-frequency situation, and needs more generation and a renewables decrease, they pay not only for their balancing energy, but also a penalty. This allows the market to allocate resources. Without this mechanism we will need to provide these resources through regulation.
Another interesting problem which arises with variable generation is the subject of decremental reserves. In the past, power plants only varied their output in an uncontrolled manner in one direction. That was down, and usually to zero.
For this, we had help from everyone on the system in the form of frequency bias to solve the problem. But wind and solar can vary their unplanned output in another direction, up. To follow NERC requirements, we now must have additional plants standing by, at low power, which can ramp up in case a unit goes down.
We must also simultaneously have other plants which are generating, and can ramp down quickly to balance load and generation in case renewables go up. Due to ramp rate considerations, these will usually be gas peaking turbines. These can rapidly decrease their output in response to renewable increases.
Without this requirement, we would be running cheaper baseload units. So the need to provide decremental reserves increases our overall cost. It increases cost in terms of units needed. But also in terms of fuel costs.
Again this cost is ignored by renewable advocates. But it is a cost. For the market to pick between renewables, it must be included.
Today, renewable providers are increasing the need for balancing services and frequency support. They are not providing them. And they are usually not paying for them. Not providing the services is not illogical.
Renewables are, on a marginal cost basis, the cheapest units on the system. They should run at a hundred percent of their capacity. They should have no headroom to provide balancing power or frequency support.
But to achieve high levels of renewables, we must explicitly consider who provides these services. We must also consider who is causing the requirements, and who pays for them. Or they will not be developed properly. We must develop the whole system.
It may seem that many small units would be less likely to cause a problem than the loss of one large plant. However, the joint probability that many small solar or wind units will decrease at once is very high, as ERCOT has proved.
If we continue down the path of using our current market rules, we will lose our future, or seriously damage it. FERC, NERC, state commissions, ISOs, etc. must work together to insure that the “Someone Else’s Problem Field” is lifted from all costs within the system.
They must ensure that those causing any cost or benefit make or receive a payment for them. So the market can make correct decisions. Our grandchildren’s future depends on it.