Ultracapacitors and batteries work together to solve power quality problems.
Chad Hall (chall@ioxus.com) is the COO of Ioxus Inc. Previously, he spent 14 years with Ioxus’ parent company, Custom Electronics Inc.
Power quality was not the important issue it is today when electrical appliances consisted of incandescent bulbs, induction motors and other devices of similar design. None of these items was overly sensitive to voltage sag, voltage swell, momentary interruptions or transients. Maybe a bulb would flicker or a motor would momentarily operate at reduced power; however, with the advent of microcomputers and sophisticated control circuitry, power quality has become important. Recent advances in storage devices such as improved batteries and ultracapacitors have made intermediate storage of electrical energy more practical and cost effective—which in turn helps utilities to deliver high-quality power at the local level.
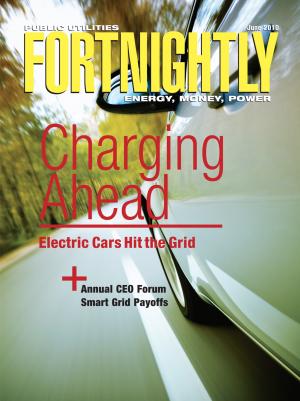
Unlike most other products, electrical energy isn’t easily stored, so when an unexpected spike in demand occurs, it often leads to a reduction in power quality and manifests in voltage sag, harmonic distortion and variations in frequency. In severe cases, excessive load can cause a low frequency, forcing a generating facility to drop off line. This can put excessive load on other generating facilities, in some cases causing a domino effect and widespread power outage. The lack of efficient storage methods requires utilities to keep a vigilant eye on demand and be prepared to bring reserve facilities into action at a moment’s notice in order to prevent power interruptions.