A new future for small coal-fired plants.
Adam Borison (adam.borison@nera.com) is senior vice president with NERA Economic Consulting. Gregory Hamm (Gregory.hamm@nera.com) is senior economist and Philip Narodick (Philip.narodick@nera.com) is associate analyst with the firm.
Small coal-fired plants are particularly vulnerable to economic and environmental pressures, putting some plant owners in what seems like a no-win position. But an emerging option—biocoal from crop wastes—might give small coal units a new lease on life.
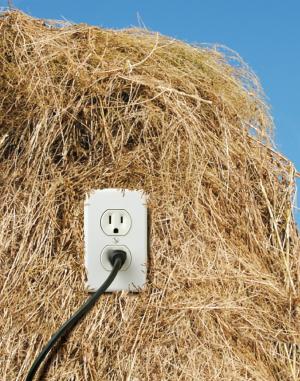
The United States has roughly 1,400 operating coal-fired generating units, producing almost 2 billion MWh of electricity a year. These units produce almost 50 percent of our electric power, along with almost 35 percent of our CO2 emissions1 and up to 40 percent of our ground-level air pollutants such as SO2 and NOx.
These 1,400 units are quite a diverse group. For example, Figure 1 shows the distribution of units by initial operation date and capacity.2 The age of these units covers a span of almost a century and the capacity covers more than three orders of magnitude from less than 1 MW to more than 1,000 MW.
Given their economic and environmental significance, there is considerable discussion both in private strategy and public policy circles regarding management decisions affecting these units. Should an older unit be shut down or refurbished? Should environmental controls be added to an uncontrolled unit? Should a unit in a suitable location be converted to natural gas? And so forth. These management decisions can have substantial impacts locally, nationally and even globally.