DER: This final installment of Oak Ridge National Laboratory's series on distributed energy resources investigates efficiency, the environment, and generation displacement.
T.K Stovall and S.W. Hadley are senior researchers at Oak Ridge National Laboratory, operated by UT-Battelle for the U.S. Department of Energy. Contact Stovall at Stovalltk@ornl.gov
Distributed energy resources (DER) have been touted as a clean, efficient way to generate electricity at end-use sites, potentially allowing the exhaust heat to be put to good use as well. However, in a time when new combined-cycle (CC) plants are being mothballed and older plants retired because of high gas prices and lack of demand, does it make environmental sense to use DER? Does DER displace other, cleaner generation technologies, or does it compete against older, dirtier power plants instead?
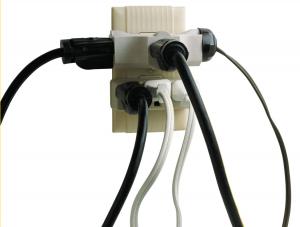
If DER systems are built, then older generating plants may be retired sooner, removing some of the worst polluters. Or it may be that DER does not directly compete against either new or old plants at the capacity planning decision-maker level. If so, increased DER simply raises reserve margins and reduces the amount of time that various central plants operate, with a mixture of results.