Elimination of the utility must-purchase obligation can lead to unanticipated consequences.
Cliff Rochlin is market advisor at Sempra Energy. Contact him at crochlin@semprautilities.com. The content hereof reflects the views of the author and does not necessarily reflect the views of the Southern California Gas Co., which has no responsibility for such content. Endnotes:
The Energy Policy Act of 2005 (EPACT) adds a new section of the Public Utility Regulatory Policies Act (PURPA) of 1978. Section 210(m) of PURPA now provides for the termination of an electric utility’s obligation to purchase energy and capacity from qualifying cogeneration facilities if the Federal Energy Regulatory Commission (FERC) finds certain conditions are met.1
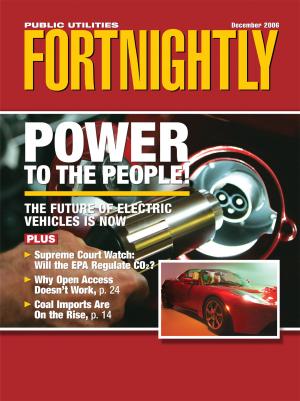
If qualifying facility (QF) cogenerators lose the utility must-purchase obligation established by PURPA, and if states do not intervene to maintain this requirement, then market conditions will dictate the amount of QF power purchased.
In this case, QF power sales become mainly a function of production costs. Since the primary function for most QFs is to provide electric and thermal energy for industrial uses for most hours of the day, power production for sale takes a secondary role. (No attempt was made to quantify other reasons for becoming a QF.) As a result, the electrical efficiency of a QF may be less than the efficiency of new, merchant combined-cycle power plants that use the waste heat to produce more electricity. If forced to compete on the cost of producing electricity based solely on the efficiency of its electric generation facilities, the QF may no longer find it profitable to operate in a baseload mode needed to meet its thermal host’s requirements.