Part 1 of a 2-part article explores new technologies most likely to influence competitive success.
David L. Bodde is on the board of directors of Great Plains Energy and is a professor of Entrepreneurship and Engineering at Clemson University.
When fighter pilots list the advantages of one combat aircraft over another, they do not speak primarily of speed. Rather, they refer to the ability of one aircraft to “turn inside” another, to negate other aspects of performance with a superior turning radius. (Figure 1 shows the contrails of the newer F-16 turning inside the vintage F-4.)
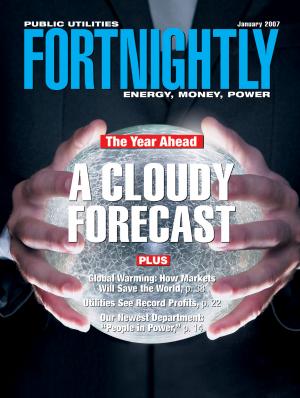
For the utility industry, fundamental changes in technology, markets, or regulatory requirements can “turn inside” the ability of companies to respond, as long-lived investments and choice of fuels lock them into their strategic choices for decades. In the meantime, such changes can expose shareholders and customers alike to unnecessary strategic risk if they are left unattended. This article proposes ways for utility leaders to understand strategic risk better and manage it more effectively.1
Part 1 of this article sets out the problem—the essence of strategic risk, its consequences for the unprepared, and the implications for utility companies. Part 2 will show how utility companies can manage, but never eliminate, strategic risk by using real options analysis and scenario planning, and by building a culture of open inquiry.