Is electricity price-elastic enough for rate designs to matter?
Ahmad Faruqui is a principal with The Brattle Group in San Francisco. He acknowledges assistance from Stacy Angel, Robert Earle, Russ Garwacki, Phil Hanser, Ryan Hledik, Roger Levy, Mike Oldak, Lawrence Oliva, Calvin Timmerman, Laura Waters and Lynda Ziegler. Special thanks to Hledik for performing the simulations described in the “Predicting Energy Impacts” section, and to Earle for insights on price elasticity simulations in general. Email the author at ahmad.faruqui@brattle.com.
Energy costs continue rising, driven largely by an unprecedented run-up in crude oil prices. As this issue went to press, crude oil futures were selling at more than $140 a barrel. Some analysts are projecting prices in the $200 range before year end.
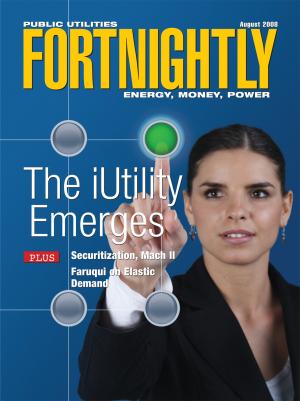
More expensive oil means more expensive natural gas, with prices now exceeding $13/Mcf. Likewise, coal prices are rising dramatically, partly in a competitive response to higher oil and gas prices, and partly in response to anticipated carbon regulation. In addition, power-plant capital costs are expected to continue rising because of growing demand in China and India for basic construction raw materials, such as cement and metals. According to one study, the cost of building new power plants is up 19 percent from a year ago and up 69 percent from three years ago. 1 Another study estimates the industry might require $1.5 trillion to meet its generation, transmission and capacity needs between now and 2030.2
Thus, in virtually every scenario, customer utility bills will rise significantly.3 Regulators and utilities are searching for ways to avoid a repetition of the rate-shock syndrome of the 1970s.