Increasing renewable generation threatens reliability.
Robert Blohm is an independent consultant active in the North American Electric Reliability Corp. for 15 years and a contributor to NERC’s Frequency Response Reliability Standard Drafting Team. Email him at rb112@columbia.edu(link sends e-mail). His webpage is http://www.blohm.cnc.net(link is external).
Obama administration energy policy targets a 500-percent increase over the next 15 years in solar, wind and tidal power’s share of the U.S. electricity system to 25 percent. If a way isn’t found to stop the North American power grids’ declining ability to respond rapidly enough to shocks, the increase in renewable generation already underway will accelerate stress on the North American power system to the breaking point.
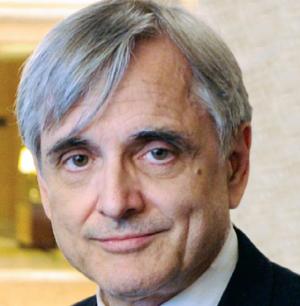
No amount of money spent on a smart grid or on expanding the transmission system will address this problem, while consequent administration pressure on the Federal Energy Regulatory Commission to fast track a solution risks backfiring into no solution at all.
Solar, wind and tidal renewables add stress to a power grid in two ways, as if in exchange for their zero fuel cost. One is by making very rapid random changes in the power they produce. The other is by their physical inability to very rapidly supply energy to arrest the effect on all generators of very rapid random generator output shortages before the effect grows too big on the power system, and thereby to help maintain a more stable continuous balance between supply and demand on the grid.