The rationale for microgrids.
Edward N. Krapels is the founder and a director of Anbaric, a project development company that specializes in large-scale electric transmission systems and small, medium, and large-scale microgrids. His article, “Busting the Transmission Trusts,” appeared in Fortnightly’s February 2013 issue. Clarke Bruno oversees Anbaric’s legal affairs and projects in the Mid-Atlantic region. He served as counsel to New Jersey Gov. Corzine, and was New York City senior attorney.
Hurricane Sandy’s impact in New York City, Long Island, and New Jersey revealed what industry experts have long known: utilities and their regulators have underinvested in the nation’s electricity infrastructure, creating a grid that is more unreliable than a modern economy needs. Sandy’s aftermath—with long delays in restoring power, communication lines out, world-class hospitals evacuating patients, sewage treatment plants dumping waste into waterways—underscored that the grid’s one-size-fits-all standard of reliability lacks justification in a world where some services are more important than others. The primacy of emergency services aside, many believe that we also need to re-evaluate how society prioritizes investment of rate-payer dollars to operate, restore, plan, and build the transmission and distribution components of the electric system.
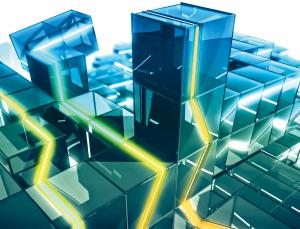
While the grid has suffered from underinvestment, the technology sector has undergone revolutions in cellular communications, microprocessor capability, and Internet connectivity. These revolutions have enabled the development of microgrids—small-scale electricity systems for one or more large users, which combine efficient generation of power with its carefully monitored use, with demand response (DR) and energy efficient technologies, in a single geographic location. These microgrids operate in parallel with or islanded from the larger grid.