Innovation must play a key role in each company.
Clark W. Gellings is vice president of innovation at the Electric Power Research Institute (EPRI). Contact him at cgellings@epri. com. Steve Hoffman is CEO of Hoffman Marketing Communications Inc. Contact him at steve@hoffmanmarcom.com.
The electric power industry is at a crossroads, and the chosen path will have significant economic and social ramifications for decades to come. The industry faces serious technological challenges in each major part of the electricity value chain—from power production to power delivery and end-use. At the same time, cross-cutting issues such as the need to increase the resilience of the power system to security threats and the ongoing need to mitigate the impact of electricity production, delivery, and use on the environment pose formidable difficulties.
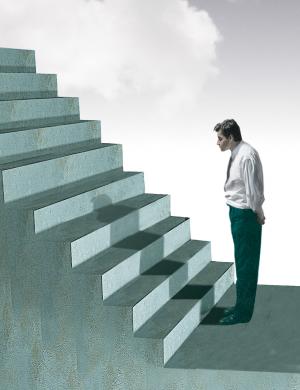
Yet, each challenge presents an opportunity. Meeting these challenges will necessitate the upgrade of our aging electricity infrastructure, which will improve the reliability and quality of power delivered to end-users. This, in turn, will improve economic productivity via reduced outage costs, fewer disruptions to sensitive equipment, and other economic benefits. Similarly, requisite advances in consumer connectivity, end-use energy efficiency, and more effective power markets will enable a range of consumer services, lower energy costs, and reduce environmental impacts. Advances in power production also promise tremendous benefits. Cleaner-burning fossil power plants will reduce emissions and sequester carbon dioxide to minimize global climate impacts, making more efficient use of resources.