What conservation potential assessments tell us about ‘achievable’ efficiency.
Dr. Hossein Haeri is a principal with The Cadmus Group. Ryan Cook, Joshua Keeling and Andrew Carollo of Cadmus assisted with the research.
Studies of energy conservation potential—known as energy efficiency potential assessments, or simply CPAs (conservation potential assessments)—can help identify and quantify gains that might come from energy conservation, namely: how much energy efficiency may be available, in which particular market segments, and when and at what cost. Such studies are essential to integrated resource planning. They serve as a cornerstone to designing effective, energy-efficiency programs. Increasingly, they are used to justify efficiency in energy policy, and serve as a basis for setting energy efficiency performance standards (EEPS). Their importance is hard to exaggerate—though not impossible—depending on how they are conducted and how their results are used.
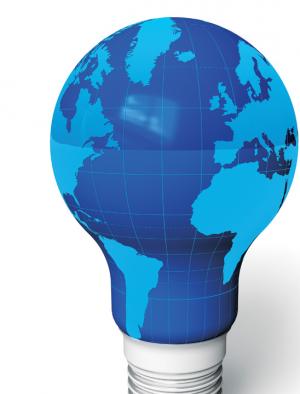
Beginnings
The roots of the CPA method can be traced to the 1978 National Energy Conservation Policy Act (NECPA). The Act, primarily a response to the energy crisis following OPEC’s 1973 oil embargo, addressed domestic concerns about energy reliability and prices, along with international concerns about economic and national security. The Act considered conservation—in the sense of using less non-renewable natural resources and increasing end-use efficiency—as one of several approaches for addressing both sets of concerns.