Price transparency will drive GHG reductions.
Fred Wellington is a managing consultant and Michael Scholand is an associate director with Navigant Consulting. This article reflects their views and not necessarily those of the COMPETE Coalition, which commissioned the white paper from which the article was adapted.
Political pressure to deliver comprehensive national greenhouse-gas (GHG) reductions is intensifying under the Obama Administration. These reductions will result from legislation, accelerated action by the Environmental Protection Agency (EPA) or both. While 2008 saw considerable debate on the structure and stringency of national GHG legislation, either Congress or the EPA (through the authority granted to it by the 2007 Supreme Court ruling in Massachusetts v. EPA1) likely will enact comprehensive GHG regulation in 2009 or 2010. The likely legislative outcome will be a market-based approach, with the cornerstone being a cap-and-trade system for CO2 emissions.
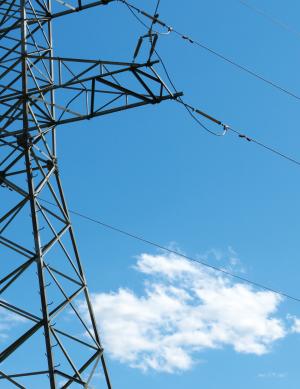
President Obama proposes to reduce GHG emissions to roughly 14-percent below 2005 levels by 2020, and to approximately 83-percent below 2005 levels by 2050. The Waxman-Markey American Clean Energy and Security Actof 2009 proposes a similar decarbonization of the U.S. economy by 2050. Together, these proposals provide a clear indication of likely future emission-reduction targets. Both proposals advocate a cap-and-trade structure as the principal policy mechanism—as do most other proposed GHG-reduction measures with similar targets for emission reductions.